Cotton Fabric Coating by rGO and Polymethylsiloxane Layer with Antibacterial, Hydrophobic and Photothermal Properties
DOI:
https://doi.org/10.48048/tis.2023.6813Keywords:
Antibacterial activity, Coating, Cotton fabric, Hydrophobic, PhotothermalAbstract
The cotton fabric, which is widely used as personal protective equipment, is vulnerable to droplets that may contain pathogens. Thus, the functionalization of cotton fabric is usually carried out to get the desired properties. Graphene-based materials have some interesting properties like hydrophobic, photothermal, and antibacterial properties that can be used to modify cotton fabric. This research has made a cotton fabric coated with graphene from coconut shells and polymethyl siloxane. Graphite was obtained from burning coconut shells, and the modified Hummers method was used to get a graphene oxide solution (GO). A graphene oxide layer on cotton fabrics was added by the dip-pad-dry process, followed by reduction using ascorbic acid to produce a reduced graphene oxide (rGO) and immersion of cotton fabrics with methyl trichlorosilane in n-hexane to produce a polymethyl siloxane (PMS) layer. Scanning electron microscope (SEM) and Fourier-transform infrared (FTIR) are used to determine the morphology and functional groups in rGO-PMS-coated cotton fabric. Contact angle measurements to determine the hydrophobicity of the rGO-PMS-coated cotton fabric, which exhibits hydrophobic properties with a contact angle of 146.3 °, which can show self-cleaning ability while preventing water droplets from entering the fabric. Antibacterial activity was carried out using the disc diffusion method against Staphylococcus aureus and delivered a good result. The rGO-PMS-coated cotton fabric also shows photothermal capability when irradiated with infrared lamps.
HIGHLIGHTS
- Graphene is a material that has various properties that can be exploited for the functionalization of ready-to-use materials
- Waste derived from natural resources can be used as a source of graphene, which can reduce the cost of graphene production
- Cotton fabric as a functionalized material can adopt the combined properties of graphene and silane such as photothermal, antibacterial, and hydrophobic properties
GRAPHICAL ABSTRACT
Downloads
Metrics
References
H Honda and K Iwata. Personal protective equipment and improving compliance among healthcare workers in high-risk settings. Curr. Opin. Infect. Dis. 2016; 29, 400-6.
S Fijan, SŠ Turk, B Neral and T Pušić. The influence of industrial laundering of hospital textiles on the properties of cotton fabrics. Textil. Res. J. 2007; 77, 247-55.
C Callewaert, ED Maeseneire, FM Kerckhof, A Verliefde, TVD Wiele and N Boon. Microbial odor profile of polyester and cotton clothes after a fitness session. Appl. Environ. Microbiol. 2014; 80, 6611-9.
T Seifi and AR Kamali. Anti-pathogenic activity of graphene nanomaterials: A review. Colloid. Surface. B Biointerfac. 2021; 199, 111509.
S Liu, TH Zeng, M Hofmann, E Burcombe, J Wei, R Jiang, J Kong and Y Chen. Antibacterial activity of graphite, graphite oxide, graphene oxide, and reduced graphene oxide: Membrane and oxidative stress. ACS Nano 2011; 5, 6971-80.
C Wang, R Guo, J Lan, S Jiang and Z Zhang. Microwave-assisted synthesis of silver/reduced graphene oxide on cotton fabric. Cellulose 2017; 24, 4045-55.
A Jishnu, SJ Jitha, S Appukuttan, AS Sethulekshmi and V Gopika. Superhydrophobic graphene-based materials with self-cleaning and anticorrosion performance: An appraisal of neoteric advancement and future perspectives. Colloid. Surface. A Physicochem. Eng. Aspects 2020; 606, 125395.
M Li, X Yang, J Ren, K Qu and X Qu. Using graphene oxide high near-infrared absorbance for photothermal treatment of Alzheimer’s disease. Adv. Mater. 2012; 24, 1722-8.
YW Chen, YL Su, SH Hu and SY Chen. Functionalized graphene nanocomposites for enhancing photothermal therapy in tumor treatment. Adv. Drug Deliv. Rev. 2016; 105, 190-204.
PC Ray, SA Khan, AK Singh, D Senapati and Z Fan. Nanomaterials for targeted detection and photothermal killing of bacteria. Chem. Soc. Rev. 2012; 41, 3193-209.
O Akhavan, K Bijanzad and A Mirsepah. Synthesis of graphene from natural and industrial carbonaceous wastes. RSC Adv. 2014; 4, 20441-8.
M Simón, A Benítez, A Caballero, J Morales and O Vargas. Untreated natural graphite as a graphene source for high-performance Li-ion batteries. Batteries 2018; 4, 13.
M Seitzhanova, D Chenchik, M Yeleuov, Z Mansurov, RD Capua and N Elibaeva. Synthesis and characterization of graphene layers from rice husks. Chem. Bull. Kazakh Natl. Univ. 2018; 2, 12-8.
EH Sujiono, Zurnansyah, D Zabrian, MY Dahlan, BD Amin, Samnur and J Agus. Graphene oxide based coconut shell waste: Synthesis by modified Hummers method and characterization. Heliyon 2020; 6, e04568.
L Sun, C Tian, M Li, X Meng, L Wang, R Wang, J Yin and H Fu. From coconut shell to porous graphene-like nanosheets for high-power supercapacitors. J. Mater. Chem. A Mater. 2013; 1, 6462-70.
AK Bledzki, AA Mamun and J Volk. Barley husk and coconut shell reinforced polypropylene composites: The effect of fibre physical, chemical and surface properties. Compos. Sci. Tech. 2010; 70, 840-6.
S Zhang, J Xu and Y Sun. Construction of porous polymer films on rGO coated cotton fabric for self‐powered pressure sensors in human motion monitoring. Cellulose 2021; 28, 4439-53.
M Shateri-Khalilabad and ME Yazdanshenas. Preparation of superhydrophobic electroconductive graphene-coated cotton cellulose. Cellulose 2013; 20, 963-72.
L Furi, ML Ciusa, D Knight, VD Lorenzo, N Tocci, D Cirasola, L Aragones, JR Coelho, AT Freitas, E Marchi, L Moce, P Visa, JB Northwood, C Viti, G Orevici, I Morrissey and MR Oggioni. Evaluation of reduced susceptibility to quaternary ammonium compounds and bisbiguanides in clinical isolates and laboratory-generated mutants of Staphylococcus aureus. Antimicrob. Agents Chemother. 2013; 57, 3488-97.
O Makarova, P Johnston, B Walther, J Rolff and U Roesler. Complete genome sequence of the disinfectant susceptibility testing reference strain Staphylococcus aureus subsp. aureus ATCC 6538. Genome Announcements 2017; 5, e00293-17.
K Zhang, Z Li, M Maxey, S Chen and GE Karniadakis. Self-cleaning of hydrophobic rough surfaces by coalescence-induced wetting transition. Langmuir 2019; 35, 2431-42.
V Angra, R Sehgal, M Kaur and R Gupta. 31 - Commercialization of bionanocomposites. In: S Ahmed and Annu (Eds.). Bionanocomposites in tissue engineering and regenerative medicine. Woodhead Publishing, Cambridge, 2021, p. 587-610.
SY Oh, DI Yoo, Y Shin and G Seo. FTIR analysis of cellulose treated with sodium hydroxide and carbon dioxide. Carbohydr. Res. 2005; 340, 417-28.
C Parida, SK Dash and C Pradhan. FTIR and Raman studies of cellulose fibers of Luffa cylindrica. Open J. Compos. Mater. 2015; 5, 52630.
B Hinterstoisser and L Salmén. Effect of fiber orientation in dynamic FTIR study on native cellulose. Carbohydr. Res. 2001; 334, 27-37.
CAR Ramírez, F Rol, J Bras, A Dufresne, NL Garcia and N D´accorso. Isolation and characterization of cellulose nanofibers from Argentine Tacuara Cane (Guadua angustifolia kunth). J. Renew. Mater. 2019; 7, 373-81.
Y Horikawa, T Itoh and J Sugiyama. Preferential uniplanar orientation of cellulose microfibrils reinvestigated by the FTIR technique. Cellulose 2006; 13, 309-16.
JD Presilla-Márquez, CML Rittby and WRM Graham. Vibrational spectra of hexa-atomic silicon-carbon clusters. I. linear SiC4Si. J. Chem. Phys. 1997; 106, 8367-73.
JP Borrajo, S Liste, J Serra, P Gonzales, S Chiussi, B Leon, M Perez-Amor, HO Yilanen and M Hupa. Influence of the network modifier content on the bioactivity of silicate glasses. Key Eng. Mater. 2004; 254, 23-6.
KY Law. Definitions for hydrophilicity, hydrophobicity, and superhydrophobicity: Getting the basics right. J. Phys. Chem. Lett. 2014; 5, 686-8.
MA Shirgholami, MS Khalil-Abad, R Khajavi and ME Yazdanshenas. Fabrication of superhydrophobic polymethylsilsesquioxane nanostructures on cotton textiles by a solution-immersion process. J. Colloid Interfac. Sci. 2011; 359, 530-5.
A Al-Jumaili, S Alancherry, K Bazaka and MV Jacob. Review on the antimicrobial properties of Carbon nanostructures. Materials 2017; 10, 1066.
K Prasad, GS Lekshmi, K Ostrikov, V Lussini, J Blinco, M Mohandas, K Vasilev, S Bottle, K Bazaka and K Ostrikov. Synergic bactericidal effects of reduced graphene oxide and silver nanoparticles against gram-positive and gram-negative bacteria. Sci. Rep. 2017; 7, 1591.
J Li, G Wang, H Zhu, M Zhang, X Zheng, Z Di, X Liu and X Wang. Antibacterial activity of large-area monolayer graphene film manipulated by charge transfer. Sci. Rep. 2014; 4, 4359.
CJ Kim, HB Kim, MD Oh, Y Kim, A Kim, SH Oh, KH Song, ES Kim, YK Cho, YH Choi, J Park, BN Kim, NJ Kim, KH Kim, EJ Lee, J Jun, YK Kim, S Kiem, HJ Choi, …, NR Yun. The burden of nosocomial Staphylococcus aureus bloodstream infection in South Korea: A prospective hospital-based nationwide study. BMC Infect. Dis. 2014, 14, 590.
M Hashemi, M Omidi, B Muralidharan, H Smyth, MA Mohagheghi, J Mohammadi and TE Milner. Evaluation of the photothermal properties of a reduced graphene oxide/arginine nanostructure for near-infrared absorption. ACS Appl. Mater. Interfac. 2017; 9, 32607-20.
A Abbas, Y Zhao, J Zhou, X Wang and T Lin. Improving thermal conductivity of cotton fabrics using composite coatings containing graphene, multiwall carbon nanotube or boron nitride fine particles. Fiber. Polymer. 2013; 14, 1641-9.
AA Balandin, S Ghosh, W Bao, I Calizo, D Teweldebrhan, F Miao and CN Lau. Superior thermal conductivity of single-layer graphene. Nano Lett. 2008; 8, 902-7.
JP Abraham, BD Plourde and L Cheng. Using heat to kill SARS-CoV-2. Rev. Med. Virol. 2020; 30, e2115.
H Zhong, Z Zhu, J Lin, CF Cheung, VL Lu, F Yan, CY Chan and G Li. Reusable and recyclable graphene masks with outstanding superhydrophobic and photothermal performances. ACS Nano 2020; 14, 6213-21.
P Kumar, S Roy, A Sarkar and A Jaiswal. Reusable MoS2-modified antibacterial fabrics with photothermal disinfection properties for repurposing of personal protective masks. ACS Appl. Mater. Interfac. 2021; 13, 12912-27.
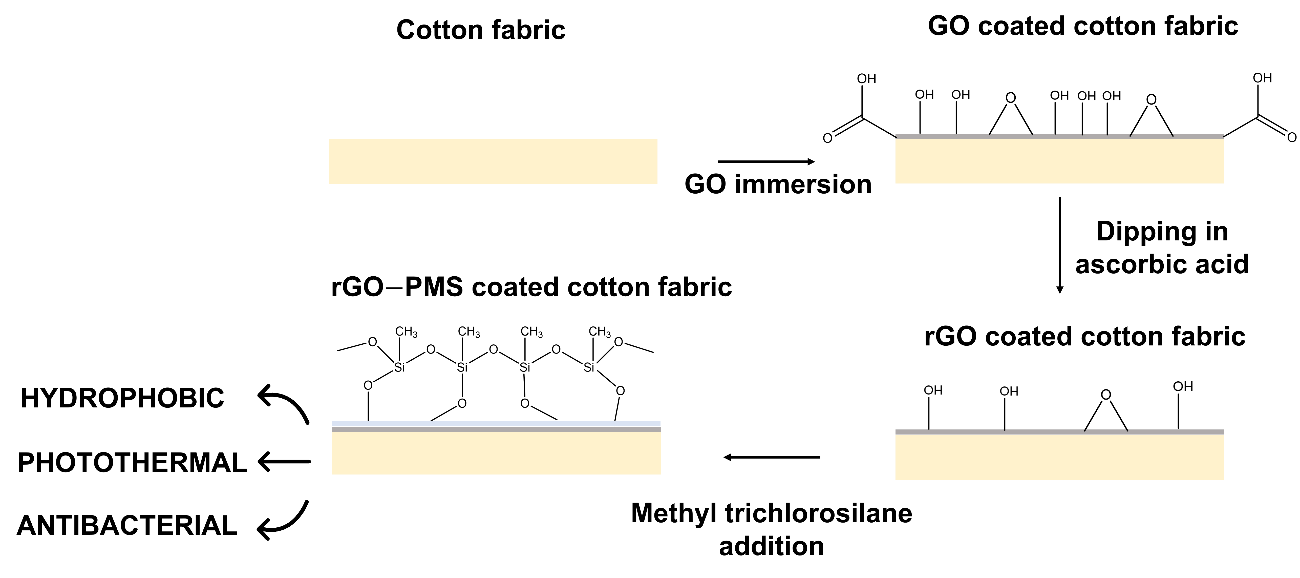
Downloads
Published
How to Cite
Issue
Section
License
Copyright (c) 2023 Walailak University

This work is licensed under a Creative Commons Attribution-NonCommercial-NoDerivatives 4.0 International License.