The Mechanisms of Neochlorogenic Acid (3-Caffeoylquinic Acid) in Improving glucose and Lipid Metabolism in Rats with Insulin Resistance Induced by A High Fat-High Fructose Diet
DOI:
https://doi.org/10.48048/tis.2023.6455Keywords:
Neochlorogenic acid, 3-Caffeoylquinic acid, Insulin resistance, Gluconeogenic genes, Glucose uptake, Lipogenic genes, AKT-AMPK pathwayAbstract
This study investigated the effects and the mechanism of actions of neochlorogenic acid (3-caffeoylquinic acid; 3-CQA) on glucose and lipid metabolism in rats fed a high fat-high fructose diet (HFFD). Male rats were fed HFFD (40 % lard and 20 % fructose) for 16 weeks. At the 10th week, the HFFD rats were split into 3 groups: HFFD receiving distilled water (HFFD control group), HFFD receiving 3-CQA 50 mg/kg and HFFD receiving metformin 200 mg/kg once daily for a further 6 weeks. At the end of treatment, fasting blood glucose (FBG), oral glucose-tolerance test (OGTT), lipid profile, insulin, leptin, adiponectin, markers of oxidative stress, and hepatic triglyceride content were measured. Liver, adipose tissue, and skeletal muscle were collected for histological, gene and protein examinations. Compared to the HFFD control group, the 3-CQA group exhibited significantly reduced FBG, insulin and leptin levels, and improved OGTT. Serum adiponectin increased and lipid profiles were normalized. Hepatic triglyceride was reduced with a decrease in lipid droplets in liver histological sections. Levels of serum SOD and CAT activity, and MDA were reversed by 3-CQA treatment. Moreover, 3-CQA significantly reduced the expression of adipocyte pro-inflammatory cytokine genes (MCP-1, TNF-α, and IL-6), hepatic lipogenic genes (SREBP1c, FAS, and GPAT), and hepatic gluconeogenic genes (PEPCK and G6Pase). Additionally, 3-CQA increased expression of muscle GLUT4 gene, and of GLUT4 protein with increased p-AKT and p-AMPK in skeletal muscle. In conclusion, 3-CQA improves glucose and lipid metabolism plausibly by decreasing oxidative stress and inflammation-induced insulin resistance, downregulating the expression of lipogenic and gluconeogenic genes, and enhancing insulin signaling in HFFD-induced insulin-resistant rats.
HIGHLIGHTS
- Neochlorogenic acid (3-CQA) improves glucose and lipid metabolism by decreasing insulin resistance
- Neochlorogenic acid (3-CQA) deceases insulin resistance due to its activities in ameliorating obesity, oxidative stress, and adipose tissue inflammation
- The beneficial effects of Neochlorogenic acid (3-CQA) to improve glucose and lipid homeostasis may be mediated via suppression of hepatic glucose production and lipogenesis, and activation of muscle glucose uptake and AKT and AMPK pathways
GRAPHICAL ABSTRACT
Downloads
Metrics
References
RN Redinger. The pathophysiology of obesity and its clinical manifestations. Gastroen. Hepato. (N Y) 2007; 3, 856-63.
RK Semple. EJE PRIZE 2015: How does insulin resistance arise, and how does it cause disease? Human genetic lessons. Eur. J. Endocrin. 2016; 174, R209-23.
MC Petersen and GI Shulman. Mechanisms of insulin action and insulin resistance. Phys. Rev. 2018; 98, 2133-23.
AG Pasavei, R Mohebbati, M Jalili-Nik, H Mollazadeh, A Ghorbani, AN Tirkani, ST Jamshidi, SI Hashemy, HH Miriand and M Soukhtanloo. Effects of Rhus coriaria L. hydroalcoholic extract on the lipid and antioxidant profile in high fat diet-induced hepatic steatosis in rats. Drug Chem. Toxicol. 2021; 44, 75-83.
DH Akhtar, U Iqbal, LM Vazquez-Montesino, BB Dennis and A Ahmed. Pathogenesis of insulin resistance and atherogenic dyslipidemia in non-alcoholic fatty liver disease. J. Clin. Transl. Hepatol. 2019; 7, 362-70.
DH Ipsen, J Lykkesfeldt and P Tveden-Nyborg. Molecular mechanisms of hepatic lipid accumulation in non-alcoholic fatty liver disease. Cell. Mol. Life Sci. 2018; 75, 3313-27.
LP Oliveira, RPD Jesús, TO Freire, CP Oliveira, AC Lyra and LG Lyra. Possible molecular mechanisms soy-mediated in preventing and treating non-alcoholic fatty liver disease. Nutr. Hospitalaria 2012; 27, 991-8.
R Marinho, RA Mekary, VR Muñoz, RJ Gomes, JR Pauli and LPD Moura. Regulation of hepatic TRB3/AKT interaction induced by physical exercise and its effect on the hepatic glucose production in an insulin resistance state. Diabetology Metabolic Syndrome 2015; 7, 67.
A Chait and LJD Hartigh. Adipose tissue distribution, inflammation and its metabolic consequences, including diabetes and cardiovascular disease. Front. Cardiovasc. Med. 2020; 7, 22.
RA DeFronzo and D Tripathy. Skeletal muscle insulin resistance is the primary defect in type 2 diabetes. Diabetes Care 2009; 32, S157-63.
G Song, Y Huang, M Xiong, Z Yang, Q Liu, J Shen, P Zhaoand and X Yang. Aloperine relieves type 2 diabetes mellitus via enhancing GLUT4 expression and translocation. Front. Pharmacol. 2020; 11, 561956.
N Liang and DD Kitts. Role of chlorogenic acids in controlling oxidative and inflammatory stress conditions. Nutrients 2015; 8, 16.
M Naveed, V Hejazi, M Abbas, AA Kamboh, GJ Khan, M Shumzaid, F Ahmad, D Babazadeh, X FangFang, F Modarresi-Ghazani, L WenHuaand and Z XiaoHui. Chlorogenic acid (CGA): A pharmacological review and call for further research. Biomed. Pharmac. 2018; 97, 67-74.
D Wianowska and M Gil. Recent advances in extraction and analysis procedures of natural chlorogenic acids. Phytochem. Rev. 2019; 18, 273-302.
AA Magaña, N Kamimura, A Soumyanath, JF Stevens and CS Maier. Caffeoylquinic acids: Chemistry, biosynthesis, occurrence, analytical challenges, and bioactivity. Plant J. 2021; 107, 1299-319.
M Imai, T Yamane, K Miyuki, S Takenaka, T Sakamoto, T Ishida, T Nakagaki, Y Nakanoand and H Inui. Caffeoylquinic acids from aronia juice inhibit both dipeptidyl peptidase IV and α-glucosidase activities. LWT 2020; 129, 109544.
CM Wu, H Luan, S Wang, XP Zhang, HT Liu and P Guo. Pandanus tectorius derived caffeoylquinic acids inhibit lipid accumulation in HepG2 hepatoma cells through regulation of gene expression involved in lipid metabolism. Yao Xue Xue Bao 2015; 50, 278-83.
DR Matthews, JP Hosker, AS Rudenski, BA Naylor, DF Treacher and RC Turner. Homeostasis model assessment: Insulin resistance and β-cell function from fasting plasma glucose and insulin concentrations in man. Diabetologia 1985; 28, 412-9.
J Ponglong, L Senggunprai, P Tungsutjarit, R Changsri, T Proongkhong and P Pannangpetch. Ethanolic extract of tubtim-chumphae rice bran decreases insulin resistance and intrahepatic fat accumulation in high-fat-high-fructose diet fed rats. Asian J. Pharmaceut. Clin. Res. 2019; 12, 506-11.
S Luangaram, U Kukongviriyapan, P Pakdeechote, V Kukongviriyapan and P Pannangpetch. Protective effects of quercetin against phenylhydrazine-induced vascular dysfunction and oxidative stress in rats. Food Chem. Toxicol. 2007; 45, 448-55.
L Góth. A simple method for determination of serum catalase activity and revision of reference range. Clin. Chim. Acta 1991; 196, 143-51.
Y Sun, LW Oberley and Y Li. A simple method for clinical assay of superoxide dismutase. Clin. Chem. 1988; 34, 497-500.
H Demir and C Demir. The comparison of antioxidant enzyme activities in quince fruit (Cydonia Oblonga) grown in van, ankara and izmir: Antioxidant enzyme activities in quince fruit grown. Med. Sci. Discov. 2019; 6, 301-4.
AR Crowe and W Yue. Semi-quantitative determination of protein expression using immunohistochemistry staining and analysis: an integrated protocol. Bio Protocol 2019; 9, e3465.
J Naowaboot, P Piyabhan, N Munkong, W Parklak and P Pannangpetch. Ferulic acid improves lipid and glucose homeostasis in high-fat diet-induced obese mice. Clin. Exp. Pharmacol. Phys. 2016; 43, 242-50.
Y Yan, X Zhou, K Guo, F Zhou and H Yang. Use of chlorogenic acid against diabetes mellitus and its complications. J. Immunol. Res. 2020; 2020, 9680508.
MF Andreoli, J Donato, I Cakir and M Perello. Leptin resensitisation: A reversion of leptin-resistant states. J. End. 2019; 241, R81-96.
SS Martin, A Qasim and MP Reilly. Leptin resistance: A possible interface of inflammation and metabolism in obesity-related cardiovascular disease. J. Am. Coll. Cardiol. 2008; 52, 1201-10.
SCDS Rosa, N Nayak, AM Caymo and JW Gordon. Mechanisms of muscle insulin resistance and the cross-talk with liver and adipose tissue. Phys. Rep. 2020; 8, e14607.
H Yanai and H Yoshida. Beneficial effects of adiponectin on glucose and lipid metabolism and atherosclerotic progression: mechanisms and perspectives. Int. J. Mol. Sci. 2019; 20, 1190.
S Hurrle and WH Hsu. The etiology of oxidative stress in insulin resistance. Biomed. J. 2017; 40, 257-62.
JG Xu, QP Hu and Y Liu. Antioxidant and DNA-protective activities of chlorogenic acid isomers. J. Agr. Food Chem. 2012; 60, 11625-30.
V Ormazabal, S Nair, O Elfeky, C Aguayo, C Salomon and FA Zuñiga. Association between insulin resistance and the development of cardiovascular disease. Cardiovasc. Diabetol. 2018; 17, 122.
L Hodson, F Rosqvist and SA Parry. The influence of dietary fatty acids on liver fat content and metabolism. Proc. Nutr. Soc. 2020; 79, 30-41.
JJ DiNicolantonio, AM Subramonian and JH O’Keefe. Added fructose as a principal driver of non-alcoholic fatty liver disease: a public health crisis. Open Heart 2017; 4, e000631.
JD Horton, JL Goldstein and MS Brown. SREBPs: Activators of the complete program of cholesterol and fatty acid synthesis in the liver. J. Clin. Investigation 2002; 109, 1125-31.
AK Min, JY Jeong, Y Go, YK Choi, YD Kim, IK Leeand and KG Park. cAMP response element binding protein H mediates fenofibrate-induced suppression of hepatic lipogenesis. Diabetologia 2013; 56, 412-22.
S Mandard, M Müller and S Kersten. Peroxisome proliferator-activated receptor α target genes. Cell. Mol. Life Sci. 2004; 61, 393-416.
KH Liss and BN Finck. PPARs and non-alcoholic fatty liver disease. Biochimie 2017; 136, 65-74.
KC Nandipati, S Subramanian and DK Agrawal. Protein kinases: Mechanisms and downstream targets in inflammation-mediated obesity and insulin resistance. Mol. Cell. Biochem. 2017; 426, 27-45.
X Huang, G Liu, J Guo and Z Su. The PI3K/AKT pathway in obesity and type 2 diabetes. Int. J. Biol. Sci. 2018; 14, 1483-96.
CA Witczak, CG Sharoff and LJ Goodyear. AMP-activated protein kinase in skeletal muscle: From structure and localization to its role as a master regulator of cellular metabolism. Cell. Mol. Life Sci. 2008; 65, 3737-55.
B Duan, Z Zhao, W Liao, H Xiong, S Liu, L Yin, T Gaoand and Z Mei. Antidiabetic effect of tibetan medicine Tang-Kang-Fu-San in db/db mice via activation of PI3K/AKT and AMPK pathways. Front. Pharmacol. 2017; 8, 535.
Y Liu, Q Wan, Q Guan, L Gao and J Zhao. High-fat diet feeding impairs both the expression and activity of AMPKa in rats' skeletal muscle. Biochem. Biophys. Res. Commun. 2006; 339, 701-7.
G Zhou, R Myers, Y Li, Y Chen, X Shen, J Fenyk-Melody, M Wu, J Ventre, T Doebber, N Fujii, N Musi, MF Hirshman, LJ Goodyearand and DE Moller. Role of AMP-activated protein kinase in mechanism of metformin action. J. Clin. Investigat. 2001; 108, 1167-74.
X Shen, L Wang, N Zhou, S Gai, X Liu and S Zhang. Beneficial effects of combination therapy of phloretin and metformin in streptozotocin-induced diabetic rats and improved insulin sensitivity in vitro. Food Funct. 2020; 11, 392-403.
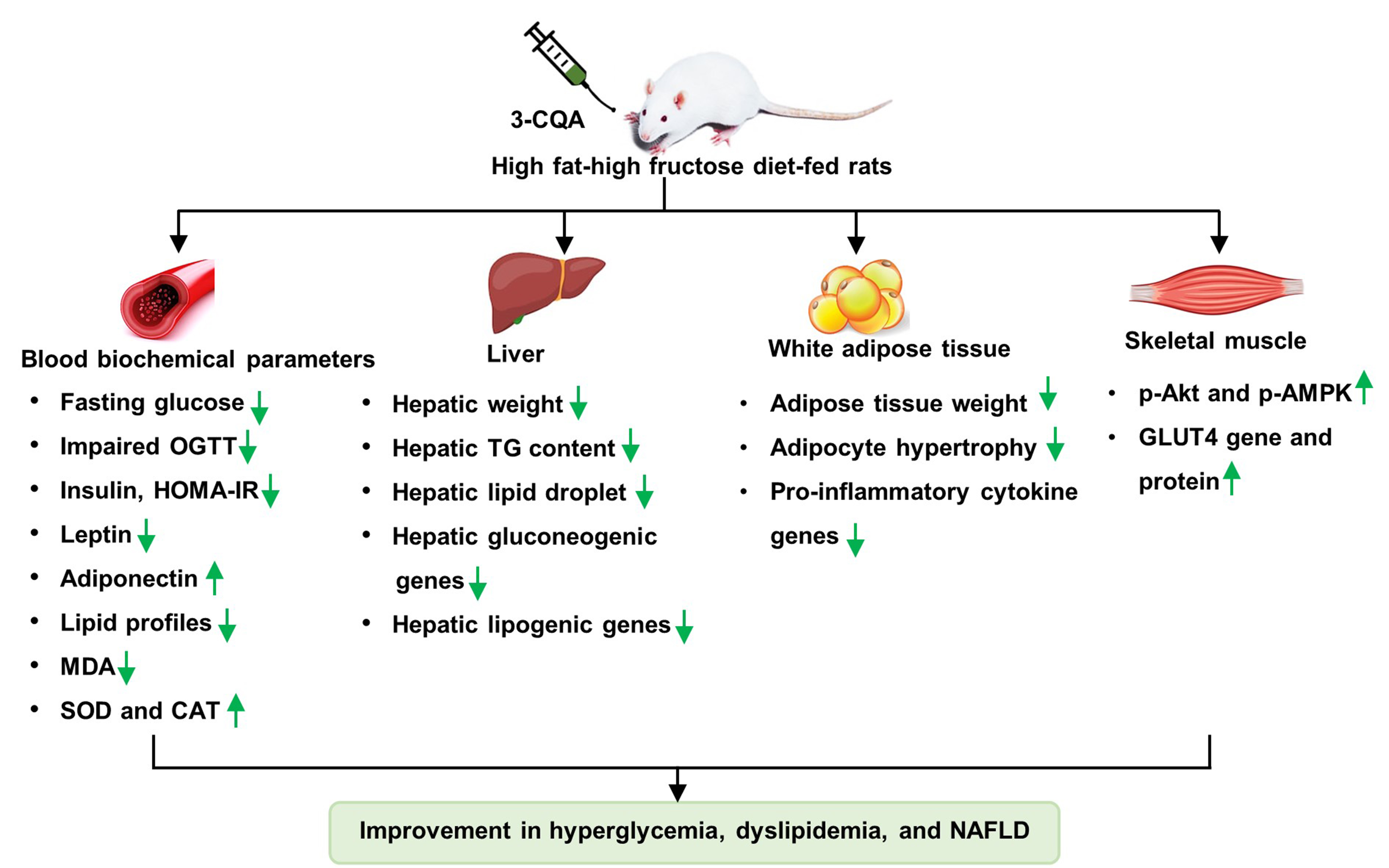
Downloads
Published
How to Cite
Issue
Section
License
Copyright (c) 2023 Walailak University

This work is licensed under a Creative Commons Attribution-NonCommercial-NoDerivatives 4.0 International License.